SLAS Europe 2021 Digital
-
You must log in to register
- Non-member - $75
- Member - $35
- Student Member - $35
- Lab Member - $35
The SLAS Europe 2019 Conference and Exhibition course package contains 29 presentations including:
26 presentations from three scientific tracks
Discovery
Emerging Biology
Technology
Three Keynote Speakers
Based on presenter permission, 26 of the 30 total SLAS Europe 2021 Digital Conference & Exhibition presentations are available on-demand.
The SLAS Scientific Program Committee selects conference speakers based on the innovation, relevance and applicability of research as well as those that best address the interests and priorities of today’s life sciences discovery and technology community. All presentations are published with the permission of the presenters.
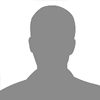
Johannes Grillari, Ph.D.
Director
Ludwig Boltzmann Institute for Experimental and Clinical Traumatology
Johannes Grillari’s research focus is on improving our understanding of the molecular and physiological mechanisms of regeneration, as well as on the impact of cellular senescence on regeneration, specifically in skin and bone. This interest has led to pioneering the field of circulating miRNAs and extracellular vesicles in aging.
Johannes Grillari has performed his studies and PhD (1999) in Biotechnology at BOKU University Vienna, Austria in the fields of cell aging and RNA biology. In 2010 he was appointed Associate Professor at BOKU, in 2019 director of the Ludwig Boltzmann Institute of Experimental and Clinical Traumatology, Vienna. He also acted as co-founder in 2011 for Evercyte, a company generating and providing immortalized cells for biopharmaceutical research and development. In 2013, he co-founded TAmiRNA, a company interested in identifying circulating miRNAs as biomarkers for aging and age-associated diseases, specifically in osteoporosis.
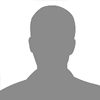
Giulio Superti-Furga, Ph.D.
Scientific Director
CeMM Research Center for Molecular Medicine of the Austrian Academy of Sciences
Giulio Superti-Furga has >25 years of experience in the use of chemical biology and omics techniques to understand drug action. He performed his studies in molecular biology at the University of Zurich, Genentech/San Francisco and the IMP in Vienna (Meinrad Busslinger).
He was a postdoctoral fellow with Giulio Draetta and Sara Courtneidge at the EMBL/Heidelberg becoming team leader in 1995. He co-founded the biotech companies Cellzome, Haplogen, Allcyte, Proxygen and Solgate. He was responsible for several large-scale projects such as genome-wide characterization of the yeast protein complexes, mapping of the entire NF-κB pathway, the viral interactome, organization of the human lipidome and global genetic interaction map of SLCs. His work focuses on molecular networks and the mechanism of action of drugs. After characterizing SLC transporters required for drugs to enter cells and other required to couple nutrient availability to mTOR activity, he became an advocate of more research on SLC transporters. He has been awarded 2 ERC Advanced grants and 2 ERC Proof of Concept grants. He is the academic coordinator of the Innovative Medicines Initiative (IMI) consortium “RESOLUTE” focusing on SLCs.
He is a steering board member (initiator) of “Genom Austria” project the Austrian Personal Genome Project initiative within the Global Network of Personal Genome Projects. His personal genome sequence PGA1 is publicly available and probably was the first full genome which was given open access to in continental Europe.
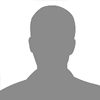
Georg Winter, Ph.D.
Principal Investigator
CeMM
Georg Winter, PhD, obtained his degree from the Medical University of Vienna, working on elucidating the mechanism of action of anti-neoplastic drugs under the supervision of Prof. Giulio Superti-Furga. He specialized on proteomics- as well as chemical genetics approaches to identify drug resistance mechanisms and synergistic drug combinations. He continued his training in chemical biology, working as a postdoctoral fellow with Dr. James Bradner the Dana Farber Cancer Institute/Harvard Medical School. Supported by an EMBO fellowship, he innovated the first generalizable pharmacologic solution to in vivo target protein degradation (Winter et al., Science 2015). He was recruited as a CeMM Principal Investigator in June 2016 where his research is now focused on using the unique molecular pharmacology of targeted protein degradation to understand and disrupt fundamental principles of transcription and gene control aberrantly regulated in human cancers. Georg Winter (co-) authored 35 manuscripts including publications in Science, Nature, Nature Chemical Biology, Nature Genetics, Elife and Molecular Cell. His interdisciplinary research lab consists of 6 Postdocs, 4 graduate students and 3 technical assistants trained in molecular biology, organic chemistry and computational biology, and is supported by several national and international grants and fellowships including an ERC Starting Grant. Dr. Winter’s contribution to the field of targeted protein degradation was acknowledged via multiple prices and awards, including the prestigious Eppendorf Award 2019 and the Elisabeth Lutz Award of the Austrian Academy of Sciences.
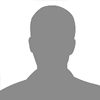
Gregory I. Vladimer, Ph.D.
Chief Scientific Officer
Allcyte
Gregory Vladimer is the CSO of Allcyte, a biotech startup in Vienna, Austria. He received his PhD from Department of Medicine at the University of Massachusetts Medical School where he studied inflammation, and was a Senior Postdoctoral Fellow at CeMM, the Center for Molecular Medicine, in Vienna. Together with the Department of Hematology of the Medical University of Vienna, spearheaded the use of single-cell image-based screening for the personalized identification of treatments for hematological cancers through a prospective clinical trial, and several retrospective studies. His work is published in journals including Nature, Lancet-Hematology, NEJM, and Cell, and he is on the steering-committee for the precision haematology working group of EHA advocating for standards around functional testing.
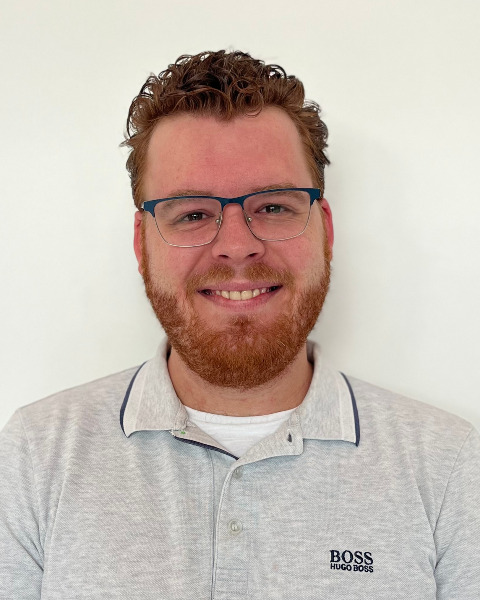
Tijmen H. Booij, Ph.D.
Lab Automation- and Screening Specialist ETH Zurich
NEXUS Personalized Health Technologies
Tijmen studied Bio-Pharmaceutical Sciences at Leiden University, during which he worked on the establishment of 3D cell culture models for drug screening. His studies were eventually awarded with the Suzanne Hovinga Award for best internship project. After his graduation, Tijmen did his PhD studies at the Leiden Academic Centre for Drug Research (LACDR) where he developed 3D cell culture-based high-throughput screening platforms for polycystic kidney disease (PKD), as well as several neoplastic disorders. His work was presented at several scientific conferences as well as several publications. Tijmen obtained his PhD degree in 2017 and is currently working as screening specialist at NEXUS personalized health technologies, a technology platform of ETH Zurich, to develop organoid-based screening methodology. Tijmen's main interests are in high-content screening with 3D tissue cultures in the context of neoplastic disorders, ultimately aiming to help discover new therapeutics.
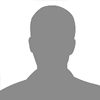
Kathrin Weidele, Ph.D.
Senior Scientist
Fraunhofer ITEM-R
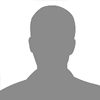
Guillaume Médard, Ph.D.
Group Leader Chemical Proteomics
Technical University of Munich
Guillaume Médard studied organic chemistry at the Ecole Nationale Supérieure de Chimie de Montpellier and the University of Strasbourg. He obtained his PhD (2009) from University College London for his synthetic work towards a natural marine secosteroid. He worked 4 years as a medicinal chemist in a drug discovery CRO in England (Argenta Discovery, now Charles River), where he specialized in kinase inhibitor discovery.
Guillaume has now been leading the chemical proteomics efforts of the Kuster lab for 9 years, at the Technical University of Munich. The key aspect of his research is the coupling of medicinal chemistry and proteomics to develop proteomics-aided drug discovery.
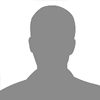
Markus Ralser
Einstein Professor of Biochemistry Head, Institute of Biochemistry
Charité Universitätsmedizin Berlin
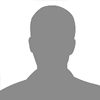
Iwan Zimmermann, Ph.D.
CSO
Linkster Therapeutics AG
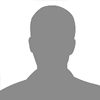
Ali Jazayeri, Ph.D.
CSO
OMass Therapeutics
Ali Jazayeri joined OMass Therapeutics in October 2018. He was previously CTO of Heptares Therapeutics, a company he joined in 2007 as one of the first scientists and was involved in the transfer of the GPCR stabilisation technology from LMB to Heptares. Following successful implementation and industrialisation of the technology, he led the development of novel methodologies that significantly increased the efficiency and reach of the technology. He was part of the team that lead the acquisition of G7 therapeutics, a spin out company from Zurich University. Prior to Heptares, Ali worked as a post-doctoral scientist in Clare Hall Laboratories and Kudos Pharmaceuticals where he carried out research on the role of cell cycle checkpoint kinases in DNA damage response pathways. He has a BSc in Genetics from University of Manchester and obtained his PhD in molecular biology with Prof Steve Jackson at the Gurdon Institute from University of Cambridge.
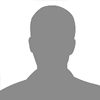
Christel Menet, Ph.D.
Chief Scientific Officer
Confo Therapeutics
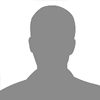
Diego L. Medina, Ph.D.
Associate Professor of Biology, Telethon Institute of Genetics and Medicine
University of Naples Federico II
Diego Medina was born in Seville, Spain. After his graduation in Biology at the University of Seville-Spain, he moved to do his Ph.D. in Biochemistry and Molecular Biology at the Instituto de Investigaciones Biomedicas/CSIC, Madrid-Spain. Then, he was awarded with a postdoctoral Marie Curie fellowship at the EMBL-Monterotondo, Rome-Italy working neuroscience using mouse genetics. Then, he joined Eli Lilly & Co as Researcher in the Lead Optimization Biology Department (Alcobendas-Madrid-Spain). In 2007 he came-back to basic research at TIGEM working in the biological mechanisms underlying lysosomal storage disorders (LSDs). Currently, Diego Medina is Assistant Investigator, Head of the High Content Screening Facility at TIGEM, and since 2019 Associate Professor of Biology at the University of Naples "Federico II". His current research interest is focused on the discovery of new ‘druggable’ targets and the development of pharmacological strategies to treat rare genetic diseases by using cell biology, high-content imaging, and OMICS.
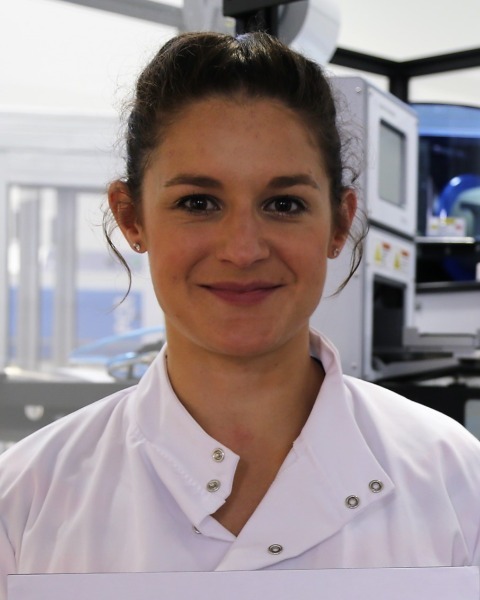
Lorna Suckling, Ph.D.
Team Leader
GlaxoSmithKline
With 13 years experience in drug discovery, Lorna has worked in both the academic andindustrial fields to gain expertise in high-throughput screening and automation. Shecompleted her PhD in Cancer Therapeutics at the Institute of Cancer Research, London followed by a Postdoctoral research position at Imperial College London where she used automated robotics platforms to develop high-throughput assays for engineering biology. Her current role at GSK includes leading a team to develop cell-based assays, in particular using High-throughput Flow Cytometry, and implementing automation capabilities for complex cell-based screening.
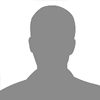
Christoph Schroder
Founder & CEO
Sciomics GmbH
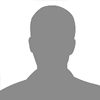
Pranav Tripathi
Postdoctoral Fellow
IIT Bombay
Pranav Tripathi is pursuing PhD in the field of aptamer based diagnostics from MNNIT Allahabad, Prayagraj, India. Under the supervision of Dr. Seema Nara, applicant has worked on novel substitutes of antibodies for diagnostic uses. Applicant is a gold medalist during his M.Tech program in Biotechnology discipline from Uttarakhand Technical University. Applicant has also published 10 research papers and 5 book chapter along with 2 patents. His area of interest lies in diagnostics especially; nucleic acid based lateral flow assays.
PhD (Biotechnology)- 2014 - till date- Motilal Nehru National Institute of Technology Allahabad
M.Tech (Biotechnology)- 2012-2014- Uttarakhand Technical University
B.Tech (Biotechnology)- 2008-2012- Amity University
CSIR-Senior Research Fellow- PhD scholar (Biotechnology)- 2014 - till date- Motilal Nehru National Institute of Technology Allahabad.
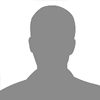
Elisa Onesto, Ph.D.
Principal Scientist, Cell Biology
Axxam
I obtained the Master Degree (Laurea Magistralis) in Pharmaceutical Biotechnologies and the PhD degree in Endocrinology and Metabolic Science at the University of Milan. I worked as Post-Doctoral Researcher at Laboratory of Neuroscience, IRCCS Istituto Auxologico Italiano In all these years the fosuc of my reserch was the identification of molecular mechanisms potentially causative of amyotrophic lateral sclerosis (ALS). I’m currently working at AXXAM as assay development scientist at research laboratories, in the field of set-up and generation of cell-based assays for High Throughput Screening
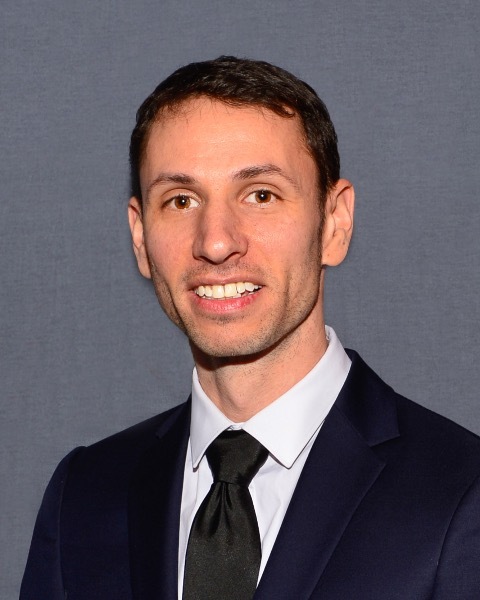
Zachary A. Gurard-Levin, Ph.D.
Chief Scientific Office
SAMDI Tech, Inc
Dr. Zachary Gurard-Levin has served as chief scientific officer at SAMDI Tech, Inc. since 2016. He brings 15 years of multidisciplinary research experience with expertise in chemistry, biochemistry, cellular biology and drug discovery research. Dr. Gurard-Levin was a pioneer user of SAMDI technology and co-developed SAMDI as a high-throughput, label-free solution for drug discovery research.
Prior to SAMDI Tech, Dr. Gurard-Levin was a research scientist at the Institut Curie in Paris, France, leading epigenetics drug discovery and diagnostics projects in oncology. Dr. Gurard-Levin has authored numerous peer-reviewed articles and has been awarded multiple research grants. He has a doctorate in chemistry from the University of Chicago. In addition, he completed a postdoctoral fellowship at Institut Curie with Dr. Genevieve Almouzni.
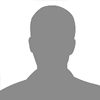
Johanna M. Kastl, Ph.D.
Senior Scientist
Origenis
I received my PhD from the University Konstanz in 2014 focusing on the identification of small molecule inhibitors of the mitotic checkpoint component Mad2. I then moved on to the high throughput screening group at Amgen. In 2016 I joined the global HTS department at AstraZeneca as a Senior Research Scientist leading HTS campaigns for several therapeutic areas. My work interests include specialist cellular assays with FLIPR or imaging readouts and their application in hit finding strategies. More recently I became interested in the implementation of protein degradation assays in screening cascades to include profiling for this MoA. I am committed to sharing my expertise mentoring and developing research scientists and students through project supervision and training.
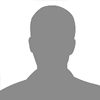
Ben Davis, Ph.D.
Vernalis
Dr Ben Davis is a Research Fellow at Vernalis Research, a biotech company based in Cambridge UK which has been at the forefront of fragment-based approaches since 1998. An NMR spectroscopist and biophysicist by training, his research focus is developing and applying biophysics and FBLD methods to enable drug discovery for challenging therapeutic targets and systems.
Dr Davis studied for his PhD in protein folding with Professor Alan Fersht at Cambridge University, and then worked on a wide range of molecular interactions in both academia and biotech companies. He has over 20 years’ experience in the drug discovery industry, has contributed to seven books over the last decade and is an author on more than thirty scientific publications. He is a frequent speaker at scientific conferences and has been running FBLD training workshops since 2007.
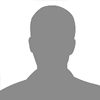
Martin Bachman, Ph.D.
Medicines Discovery Catapult
Martin obtained his MSci degree in Medicinal Chemistry from University College London, followed by a CRUK-funded PhD in Chemical Biology and Molecular Medicine in the groups of Sir Shankar Balasubramanian and Dr Adele Murrell at the University of Cambridge. During this interdisciplinary project, Martin combined chemical synthesis of stable isotope labelled probes with cell culture, in vivo experiments and mass spectrometry to address unanswered questions in the field of epigenetic modifications of nucleic acids. He then moved on to an industrial postdoc position at AstraZeneca where he worked on the development of Acoustic Mist Ionisation high-throughput mass spectrometry platform in collaboration with Waters and Labcyte. In 2018, Martin joined the Medicines Discovery Catapult as a Lead Scientist to apply innovative mass spectrometry-based solutions to drug and biomarker research.
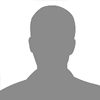
Adrien Pasquier, Ph.D.
Post Doc
AstraZeneca
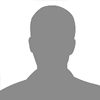
Ola Engkvist, Ph.D.
Head Molecular AI
AstraZeneca R&D
Dr Ola Engkvist is head of Molecular AI in Discovery Sciences, AstraZeneca R&D. He did his PhD in computational chemistry at Lund University followed by a postdoc at Cambridge University. After working for two biotech companies he joined AstraZeneca in 2004. He currently lead the Molecular AI department, where the focus is to develop novel methods for ML/AI in drug design , productionalize the methods and apply the methods to AstraZeneca’s small molecules drug discovery portfolio. His main research interests are deep learning based molecular de novo design, synthetic route prediction and large scale molecular property predictions. He has published over 100 peer-reviewed scientific publications. He is adjunct professor in machine learning and AI for drug design at Chalmers University of Technology and a trustee of Cambridge Crystallographic Data Center.
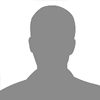
Christopher Southan, Ph.D.
Competitive Intelligence Analyst
Medicines Discovery Catapult
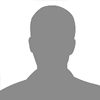
Daniel A. Thomas, Ph.D.
LCGI Head of Discovery Biology
Arctoris Ltd
Daniel Thomas is Head of Discovery Biology at Arctoris where he is jointly responsible for the implementation of a comprehensive scientific strategy designed to deliver high quality, reproducible and richly annotated data sets supporting early research. Blending the latest in biochemical, cellular and molecular techniques together with cutting edge automation and data analytics, the goal is to deliver automated pipelines to eliminate unnecessary experimental variability. He received his BSc and Ph.D. from the University of Leeds before joining GlaxoSmithKline, where he spent 20 years working in small molecule drug discovery. He has a passion for enzyme kinetics, extensive theoretical and practical knowledge of assay development and is an accomplished leader. He has been responsible for the quality oversight of substantial production screening portfolios, overseen the delivery of mechanistic data across multiple therapeutic areas and was principal industrial contributor in the collaborative development and implementation of a number of transformative technologies.
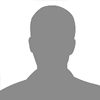
Tobias Brode
Head of Business Unit, Medical Engineering and Biotechnology
Fraunhofer IPA
Tobias works in various roles at the Fraunhofer IPA on the subject of laboratory automation. As a business unit manager, he is passionate about building bridges between research and industry and applying new technologies.
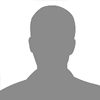
Sebastian Bierbaum, M.S., Ph.D.
Student Enabling Technologies
Bayer AG
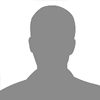
Thomas Huser, M.S., Ph.D.
Professor of Physics
University of Bielefeld
Thomas Huser is a Professor of Physics at the University of Bielefeld, Germany. He holds several patents on single molecule and single cell analytics, as well as super-resolution microscopy, and has over 120 peer-reviewed publications. From 2005 - 2011 he was an Associate Professor in the Department of Internal Medicine at the University of California, Davis, and also served as Chief Scientist for the NSF Center for Biophotonics Science and Technology. From 2000 - 2005, Dr. Huser was a Group leader for Biophotonics and Nanospectroscopy at Lawrence Livermore National Laboratory in Livermore, CA, where he developed and applied novel nano-biophotonics tools to the analysis and characterization of individual cells. Dr. Huser obtained his Ph.D. in Physics from the University of Basel, Switzerland, on near-field optical microscopy. At the University of Bielefeld he applies optical nanoscopy and spectroscopy techniques to biological and medical problems at the single cell level.
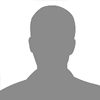
Pilar Ayuda-Durán, Ph.D.
Postdoctoral Researcher
Oslo University Hospital
I’ve always been interested in cancer research. My PhD work was about the effects of an altered cell cycle misregulation in the occurrence of genomic instability, a common feature in the origin of tumour development. For that purpose I worked with the useful model system Saccharomyces cerevisiae. After obtaining my PhD I decided to move to a disease-related research. Jorrit Enserink provided me the opportunity to combine basic research using a leukaemia model (mice Ba/F3 cells expressing the BCR-ABL oncogene, common in CML and ALL) with a more clinical-related challenge, the establishment of a personalized medicine platform. Altogether these research paths fulfil my desire of contributing to unravel cancer biology and helping to design new treatments against this disease.
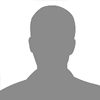
Christian Brüser, Ph.D.
Post-Doc
Max-Planck-Institute for Biophysical Chemistry
I studied Biologie at the Heinrich-Heine University in Düsseldorf. During my Master‘s studies I worked intensively with superresolution microscopy and analyzed the degradation of transmembrane receptors through the endosomal pathway. In 2014, I joined the laboratory of Prof. Stefan Jakobs at the Max-Planck-Institute for Biophysical Chemistry in Göttingen. I received my PhD in 2018 and since then I have been working as a Postdoc, investigating the behavior of mitochondrial DNA and the organization of the inner mitochondrial membrane, using a variety of superresolution microscopy techniques, especially STED-microscopy.
Key:




